Adapting an experimental tool for use in a new field can be as powerful as inventing a new technique.
Physics is an empirical discipline, so although theories are vital for conceptualization and for suggesting interesting avenues to explore, their proof is always in the experimental pudding. This means that any time that a new experimental tool becomes available there is justifiable excitement about what its use might reveal. One way this can happen is when researchers realize that methods that are established in one field can be ported to another. In this issue of Nature Physics, we showcase three subfields where this has recently occurred.
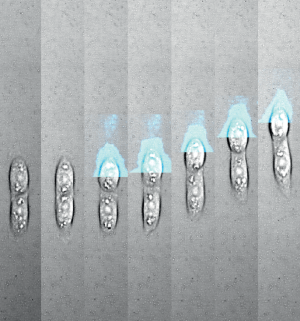
High-harmonic generation is a nonlinear optical process where a target can emit photons at integer multiples of the frequency of an irradiating laser pulse. First observed in atomic gases and a fundamental aspect of attosecond science, it has more recently been employed as a spectroscopic tool to investigate the electronic physics of quantum materials. In their Review, Christian Heide and colleagues describe how the microscopic details of high-harmonic generation depend not only on the electronic band structure of the material in question, but also on other specific characteristics such as the presence of surface states or strong electron–electron interactions.
For example, although centrosymmetric materials can typically only generate odd harmonics, experiments on topological insulators show strong even harmonics that originate from the surface states. More detailed investigations of these materials — for example, of the dependence of the high-harmonic generation process on the ellipticity of the driving laser — can highlight the topological phase transition itself. Or, because strong interactions between the electrons can modify the band structure, temperature-dependent measurements can reveal the emergence of strongly correlated states as the sample is cooled. However, the community has not yet developed a rigorous theoretical understanding of the mechanism of high-harmonic generation in this regime, leaving many open questions to be resolved.
In an Article in this issue, Charlotte Bøttcher and co-workers use a circuit quantum electrodynamics approach to probe the superfluid density of a superconductor–ferromagnet heterostructure. Circuit quantum electrodynamics has its roots in the study of the interaction of light within superconducting circuits and is the main method for controlling and interrogating superconducting qubits. It operates by channelling microwaves to the qubit to engineer strong coupling between the photons and the device. But other quantum materials can replace the qubit, and the combined response of the strongly coupled system can say something about the material’s properties.
The heterostructures that Bøttcher and colleagues investigated are the focus of much attention, owing to their potential to host topologically protected excitations that exhibit non-Abelian exchange statistics. These quasiparticles are interesting on a fundamental level, but also because of their prospective applications in quantum computing. However, the exact symmetries of the superconducting state — and hence whether it can indeed host topological excitations — are still not fully known. The team extracted the superfluid density and found a two-fold spatial anisotropy that indicates a p-wave symmetry, and a temperature dependence that suggests the superconducting state is not fully gapped. This is further evidence that these heterostructures may have the superconducting properties required to host non-Abelian quasiparticles.
Last but not least, optogenetics has recently arrived as a tool in the realm of biophysics. This technique uses genetic engineering to design and incorporate light-sensitive proteins inside the cell. Their biological function can be precisely tuned through optical modulation, enabling the control of specific cellular functions. It has been used extensively since the early 2000s in neuroscience and even has medical applications, for instance in treating cardiac diseases.
Leone Rossetti and collaborators applied this technique in their Article to create so-called leader cells that guide cell migration during processes such as wound healing and cancer growth. They did this by using blue light to activate a protein known as Rac1 that distinguishes the leader from the follower cells. Once the leaders were created, they could measure the traction forces and tension propagation in cell clusters with increasing size. Intriguingly, the team found that the leader cells could effectively drag one follower cell (pictured as a time series), but not larger clusters. This is in contrast to the established picture that leader cells are autonomous.
These examples show how experimental methods can be moved from one discipline to another to address new problems and provide insights that will no doubt spur the next iteration of discoveries.